- Seebeck | Peltier | Thomson | Joule effects
- Generate electricity thanks to chemistry
- Alternative solution: solar and photovoltaic panels
- Quiz
Generate electricity thanks to chemistry
Ultra-confined liquids or Solid thermoelectric
Solid thermoelectric materials convert a temperature difference into electrical energy. They are thus an important energy resource for years to come. However, the most efficient materials are rare, expensive and often toxic.
However, an alternative possibility exists and consists in the use of nanofluidic channels confining salt water. Such systems have attracted much attention recently, as they are capable of producing electricity from the osmotic energy of seawater. This «blue energy» comes from the phenomenon of osmosis, that is, the spontaneous flow of the liquid from the most concentrated medium to the least concentrated. But the application of these devices to the electricity recycling of waste heat from many industrial processes in electricity is only now beginning to be studied. This lower interest can be explained by the standard image of the thermoelectricity of charged liquids, developed in the 1980s, which predicted performances far below those of thermoelectric materials.
This original approach, transposable to materials of very varied shapes and natures, provides a theoretical framework to predict the unusual behaviour of charged liquids, especially in contact with nanoporous metal structures, with direct applications in the field of energy, environment or chemistry.
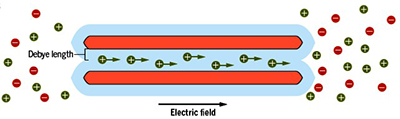
How does it works?
By circulating liquids in charged nanoscale channels, it is possible to convert heat into electricity as efficiently as the best thermoelectric materials.
The scientists tested these models by simulating the behaviour of the material at the atomic level. In this type of simulation, the motion of each atom is described explicitly, which makes it possible to measure independently the influence of the various parameters (interactions with the walls, electrostatic contribution) on the movement of atoms and therefore of the electric current. Against all odds, they showed that the performances of nanofluidic systems were a hundred times higher than the predictions of standard models, and could be comparable to those of the best solid thermoelectric materials. This work demonstrates the potential of nanofluidic systems and, by helping to understand their mechanisms, can serve as a guide for the development of high-performance devices, an economical and non-toxic alternative to thermoelectric materials.
When subjected to a temperature difference, a nanofluidic channel can generate electricity, with a yield comparable to that of the best thermoelectric solids.
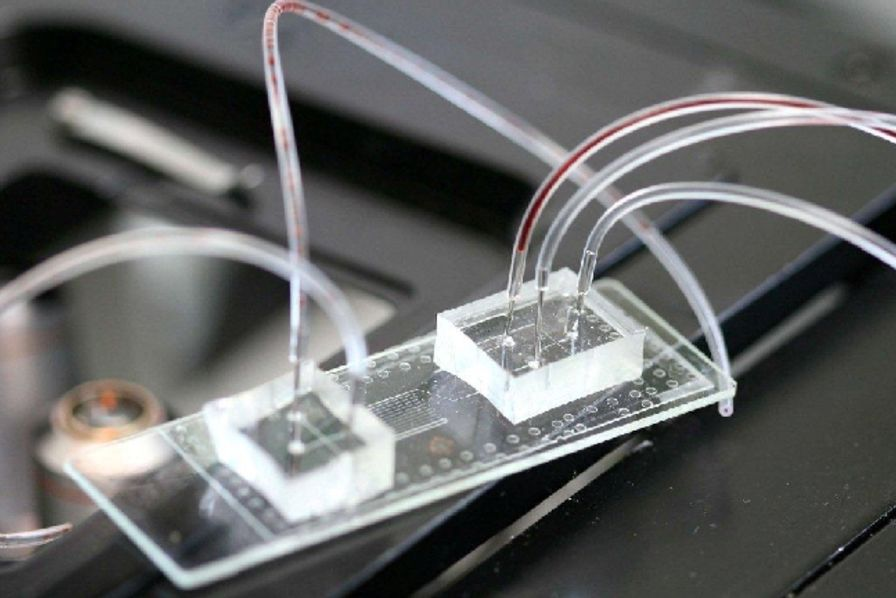
About the future
Scientists have introduced a new atomic-scale simulation method that describes the containment or adsorption of a charged fluid near a surface, taking into account the effects of electronic relaxation, in other words, the movement of electrons towards electric charges approaching the material. The approach consists in mimicking the effects of electron clustering against the charges of the liquid – the electrostatic screen – for any situation between an insulating material and a perfect metal. For this, Coulomb interactions are described via a “virtual” Thomas–Fermi fluid made of light and fast charged particles that imposes an electrostatic screen by reorganizing in the presence of the confined liquid. Using this strategy on a nano-confined ionic liquid, there is a wetting transition depending on whether the confining material is insulating or metallic.
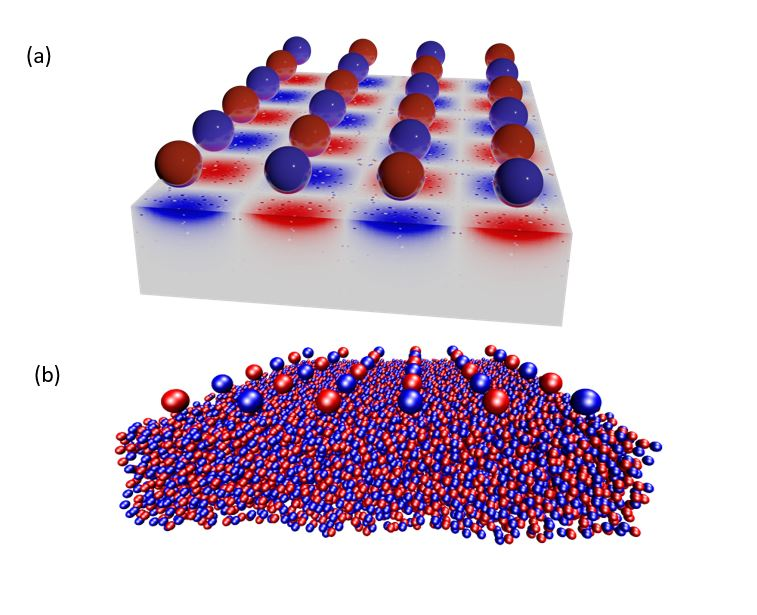
Used in energy storage, electrochemistry and catalysis, liquids with polar ions or molecules exhibit unexpected behaviours in the vicinity of a solid surface, especially when confined. For example, there is an unexplained link between the solidification temperature of the charged liquid and the metallic or insulating nature of the surface. The complexity of electrostatic interactions in confined geometry is a more active research topic than ever, but despite this, the modelling of ionic fluids at the interfaces remains partial and some of the observed behaviours still escape theoretical understanding. While most approaches assume perfectly insulated or metallic surfaces, the difficulty is to simulate the reaction of a surface in the case of real materials, whose electrostatic response is mostly intermediate between insulation and metal.